20 Pages
5056Words
Introduction Of Protein Mutation In Sickle Cellanaemia Assignment
Need assistance with assignment writing in the UK? New Assignment Help has got you covered. Our skilled writers craft well-researched assignments tailored to your specifications.
SCD refers to a genetic disorder that is caused due to mutation in a person’s HBB gene. The HBB gene has been involved in encoding haemoglobin components, a protein that carries oxygen in red blood cells (RBC). The mutation leads to causes the molecules of haemoglobin to stick together, therefore, develop a sickle-shaped red blood cell. Cell blood becomes ruptured and recurring pain, anaemia, and organ damage, and immunodeficiency, leads to early death. SCD is gradually becoming a severe monogenic disorder across the world. SCD prevalence is reported to be high in India, sub-Saharan Africa, the Middle East and the Mediterranean parts. Studies show that the prevalence of SCD is high in India (South Asia) where 20 million SCD patients live. The data has been gathered through village-level prevalence surveys and State-wide screening programmes that focus on groups and βS allele high prevalence.
Protein mutation mechanism
Sickle cell anaemia is a condition known to be caused by the occurrence of mutations in the beta-globin (HBB) gene, which cause the population of an abnormal version of subunit of the haemoglobin which is the protein which takes responsibility for carrying the Oxygen in the RBC. After the mutation occurred, the "mutable protein" is known to be haemoglobin S (Caoet al.2021). The mutation makes the allele different from the wild-type β-allele causing a nucleotide polymorphism dbSNP Rs334, which is responsible for the substitution of the GTG codon with by the GAG codon in the beta-globulin gene. It leads to the hydrophilic glutamic acid residue replacement with a hydrophobic valine residue (Val) in the β-globin chain. It results in haemoglobin tetramer HbS (α2βs2) mutation in the individual's erythrocytes SCA. Homozygous inheritance of the coinheritance of βS or βS mutation (HbSS) with other mutations which includes βD (HbSD), βC (HbSC), βE (HbSE), βO (HbSO/Arab), leads to other forms of SCD through cellular mechanisms and multiple interlinked molecular. This disease when occurs, blocks the flow of blood to the organs and causes the affected organs to lack oxygen, and makes it deprives them of blood. In the patient who is suffering from sickle cell anaemia, the blood is low in oxygen (Demirciet al.2019). The blood which is lack oxygen could cause damage to the organs and the nervous system, which would damage the organs which include the liver, spleen, and also kidneys, which could be fatal for any patient.
Sickle Cell anaemia is a genetic disorder affecting the production of haemoglobin, the protein within the blood responsible for carrying out oxygen. Haemoglobin is made up of four subunits of protein, each containing a heme molecule which binds the oxygen. In the case of sickle cell anaemia, a single amino acid substitution occurs in one of the protein subunits of haemoglobin, replacing the normal glutamic acid with a valine (Sunddet al., 2019). Due to the change in a single amino acid, the structure of the haemoglobin changes and causes the protein to aggregate, forming rigid, long and sickle-shaped structures in the red blood cells. According toAl-Janabiet al.,(2021), the condition is caused due to a single-point mutation in the beta-globin gene that is in the beta-S (βS) allele which is located in the 11p15, 5-chromosome region. As stated byCarden et al., (2020), a systematic evaluation of Global Burden of Disease Study depicts that around 3.2 million people are suffering from sickle cell anaemia with43 million individuals processing the trait of the sickle cell which indicates that they are the carriers of mutation. It has been also found that176,000 people face death per year due to sickle cell anaemia-related complications.
Figure 1: Suckle cell anaemia- Molecular pathophysiology
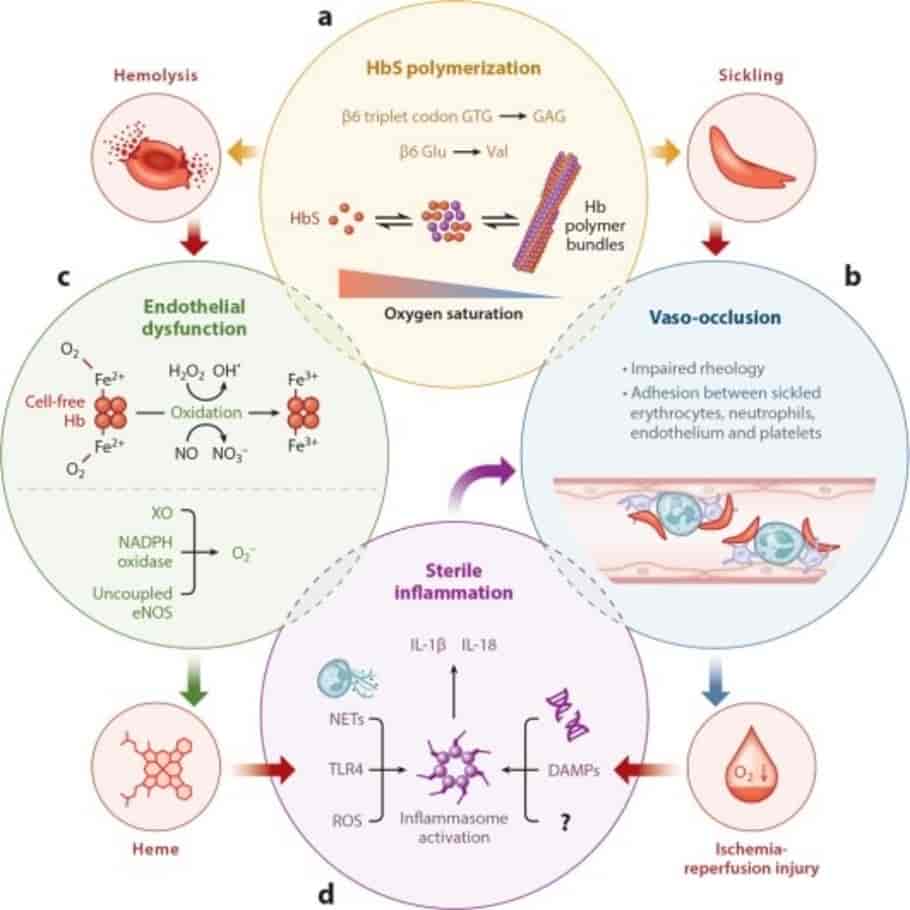
The nucleotide polymorphism, replacing the hydrophilic Glu (Glutamic acid residue) with a hydrophobic Val (valine), results in mutated haemoglobin tetramer HbS (α2βs2) in the RBC, which reduces the flexibility of the cell with a shorter lifespan which leads to a reduced oxygen-carrying capacity of the blood Sickle cell anaemia has been used as an umbrella term for all the mutations occurring within the β-globin gene, homozygous inheritance of the βSmutation (HbSS) to coinheritance of βSwith other types of mutations which include βD (HbSD), βC (HbSC), βO (HbSO/Arab), and βE (HbSE) (Yousif, 2022). These mutations cause different forms of sickle cell anaemia by multiple interlinking cellular and molecular mechanisms.
Evidence of disease referring to the current molecular knowledge
Sickle cell anaemia is a disease that affects the haemoglobin protein and causes issue with the delivery of oxygen to cells. The mutated protein formed by the disease is the haemoglobin S, which distorts the shape of the red blood cells. The evidence of the disease is that the patient has a major symptom of periodically occurring episodes of pain crisis (Martinset al. 2023). The pain occurs when there is a blockage of the tiny vessels of blood in the joints of the hands and legs, chest, and other areas of the body like the abdomen. The intensity of the pain could have a lasting from a few days to a few hours. Normal haemoglobin is a protein that when mutated causes sickle cell anaemia. It differs from the mutated gene by only a single amino acid- The normal haemoglobin has an amino acid which is named Valine, which is replaced by glutamate amino acid at the 6th position on the beta surface chain (Bhaskaret al.2019). The molecular weight of haemoglobin A which is the most dominant form of haemoglobin in adults is 64400. Sickle cell anaemia could be identified in a patient by doing blood tests and tests for genetics. Genetic tests are the more accurate form of tests that gives accurate results for the consequences and whether there are one or two or more copies of the sickle cell genes in the body.
The sickle solubility test is the most common screening technique which is currently the most relevant; the other techniques include isoelectric focusing (IEF), high-performance liquid chromatography (HPLC), and haemoglobin electrophoresis. However, many of the screening tests have their advantage and disadvantage (Jiet al.2020). The low-cost screening techniques include the sickle solubility test, which is the insolubility of Hbs in the presence of certain agents that acts as reducing agent in nature like "sodium dithionite", by turbidity detection of the formation of the crystals from the breakage of Hbs- containing Erythrocytes (Wareet al.2021). These techniques or screening tests could provide the qualification of the haemoglobin relatively, and the differentiation of the cells. Certain rare haemoglobin traits combine thus the use of certain gel bases like cellulose acetate and the citrate agar or the "IEF methods" is often required for the further discrimination of the haemoglobin.
Current Therapies and Their Evidence
The research evidence which is latest on sickle cell anaemia disease is that the researchers have proved a link or relation between Hypoxia and Fetal Haemoglobin which provides evidence hopefully for the disease. On 12th October 2022 - the studies have shown evidence to the response was at the cellular level, having a low level of oxygen which in return also causes an increase in the expressions of fetal haemoglobin in the adults, which would cause the treatments of the genetics of the human body (Adioet al.2022). The new experimental therapy that has successfully resulted in the cure of the disease is called LentiGlobin, which is a gene therapy where the doctors harvest' the stem cells of that patient's body and add them to the haemoglobin cells which are non-sickle in the corrected gene. Dr. Rifkin- Zenenberg, is the investigator who is the principal, and the main person who focussed on this experiment for the gene therapy study.
In the healthcare sector, physicians treat this disease in several ways like a transfusion of blood, treatment with antibiotics, and other medications which reduces the complications of sickle cell anaemia in any patient's body. The medications might include voxlelotor, hydroxyurea, crizanlizumab and L-glutamine therapy. Voxlelotor is used to treat SCD among children and adults who help in holding the haemoglobin into more oxygen. Hydroxyurea enables the RBC bigger which helps the cells to be round and flexible. It ultimately prevents them to turn sickle-shaped. Crizanlizumab has been proposed to reduce the pain of an individual due to SCD. It allows the blood cells to prevent from getting stick to the blood vessel from inside. L-glutamine therapy in SCD patients lowers the damaging rate which causes due to blockage by blood cells (Penkertet al.2021). However, the current therapy for the cure of sickle cell disease is the Blood and Bone marrow Transplant (Abdulwahabet al.2021). The healthcare sectors always recommend early diagnosis, for which they could do early treatment by recommending proper medicines to manage the complications including pain which is chronic. Early diagnosis for the identification has been introduced for SCD however; cure and treatment are still under process. Also, regular check-ups should be taken care of for the carrier patients.
New therapies and their pieces of evidence are proven in the current scenario for the patients suffering from sickle cellanaemia Crinzanlizumab takes an approach that is different and modern in certain ways supporting the system of blood circulation in the person's body. It is a therapy that focuses on the improvement of the haemoglobin in the RBC, where it makes the blood vessel walls of different organs less sticky, preventing the sickled cells from getting clotted together and altering the flow of the blood. This therapy would have a better scope in the future as, the blood vessels could be modified according to the situation of the RBC, making them smoother and easier the flow. It is always necessary to think of or innovate another procedure or medical therapy if one does not solve the problem.
The National Institute of Health advises that patients suffering from SCD would be provided with healthcare facilities and several treatment opportunities which would help in the specialization of SCD. All the patients would have the opportunity to consult with the hematologist frequently. The sickle cell defect could be solved more technically and scientifically by using gene editing or the addition of genes using lentiviral vector-based strategies or Hb F induction (El Hosset al.2020). The strategy for the gene addition has met the clinical trials and proven them to be successful where the stem cells of the patient's body are infected using a lentivirus which expresses an anti-sticking β-globin variant, T87Q. The vector helps in the substitution of the (βA–T87Q) (Neaet al.2021),which would allow high performance in the liquid chromatography (HPLC) which helps in the monitoring of the globin levels of transgene in the cells of the patient.
The induction of Hb F is a well-established process that increases the efficiency of HbF that has motivated the pharmacological and the approaches for the genetic ones to the HbF induction. Gene editing is another successfully proven method that rectifies the sickle cells of three patients, it corrects the defective DNA in its original location. Certain strategies are used for the gene editing procedure, like transcription activator-like effector, clustered regularly interspaced" short palindromic repeat (CRISPR), nucleases (TALENs) and ZFNs, and the associated with the Cas9 nuclease (Germino-Watnicket al.2022). This technology has an approach where it makes double-stranded bread in the sequence of a particular gene that guides directly to the RNA.
The disease has proven to be fatal in patients if not properly diagnosed. Negligence in the treatment has caused cases to worsten in certain scenarios (Menzeet al.2019). Although the complete care of the disease is not possible, as it is a genetic disease and negligence of it could lead to drastic results in the future. It has been seen that improper diagnosis caused late medication and treatment of the patient which made them suffer. Thus, if early diagnosis could be done for the patient using any of the screening methods or technologies, would help the patient to recover faster and have a better lifestyle in the future. Further studies and references are being researched and it has been expected that certain cases would develop which would help to cure the disease permanently and also be cost-effective for the human race, providing them with a better future (Haro-Moraet al., 2020).
Suggestions on future approaches
A wide knowledge of the molecular, biophysical and cellular nature of Sickle cell anaemia has currently provided many potential and significant therapeutic approaches for the future which aim at reducing and preventing the disease. It has been found that the current therapies attenuate the severity of the disease by impeding the different pathobiological facets of sickle cell anaemia (Uchidaet al., 2021). Some significant therapies focus on the prevention ofHbS polymerization as well as rescuingerythrocyte deformability by influencing the production ofHbF. Other treatment focuses on the modification of HbS oxygen affinity allosterically along with the prevention oferythrocyte dehydration. Currently, antisickling therapies or anti polymerization therapies, and variousantiadhesion therapies have been approved for the treatment of sickle cell anaemia which focuses on inhibiting the cascade ofmulticellular adhesion in the vaso-occlusion (Chu et al., 2020). It has been focused that these therapies are typically directed at E-selectin (rivipansel), P-selectin (crizanlizumab), Mac-1 (intravenous immunoglobulin), MEK inhibitors or platelet glycoprotein Ibα (CCP-224) which aim at the prevention oferythrocyte adhesion.
Figure 2: Suckle cell Anaemia- Current and Future Therapies
-min_65facaefd3b71.jpg)
CRISPR technology
A substantial and rapid progression in the approaches of genome editing has proven potential as a curative and preventable option for sickle cell anaemia which aims at either correcting the underlying mutation in thehematopoietic progenitor cells or stem cells in the patient or inducing fetal expression of haemoglobin to overcome the sickling of the red blood cells or creating corrected pluripotent stem cells by different approaches (Haro-Moraet al., 2020). The occurrence of CRISPR/Cas9 has been a revolution in the field of “genome engineering as well as brought various clinical treatments for various diseases. It has been thatCRISPR-Cas9 technology as a powerful gene-editing technology has the potential for the treatment of various “genetic disorders” which includes Sickle cell anaemia as well.
The technology utilises an RNA guide for targeting and cutting a specific sequence of DNA, which allows forprecise editing of the genetic code. CRISPR can be used as a gene-editing tool toedit the faulty gene responsible forthe production of abnormal haemoglobinwhich causes sickle cell anaemia (Vendrameet al., 2019). The technology can be used to make precision changes in theDNA sequence of the HBB gene to correct the mutation and restore the production of normal haemoglobin by creating protective elections and insertions. In this process, programmable nucleases have been utilised for the creation of DNA double-strand breaks (DBS) at a superficial locus of the genome and then DNA repairing mechanism has been employed by non-homologous end-joining or homology-directed repair (Tisdaleet al., 2020)
Figure 3: Application of CRISPR Technology for the treatment of Sickle cell disease.
-min_65facb15c3f0a.jpg)
Previously, three significant nucleases have been utilised for genome editing processes which include meganucleases, zinc finger nucleases and TAL-effector nucleases which helped to successfully correct the mutation of SCD and induce fetal globin through editing the regulatory sequences which include promoter sequences or KLF1, BCL11A and MYB to overcome the mutation severity in the patients (Nkyaet al., 2020). The application ofCRISPR/ Cas9 technology has been proven most effective due to its high efficacy in correcting the mutation in SCD and the high inducing capability ofHbF expression. Cas9 cuts the sickle beta-globin and repairs it with the normal beta-globin sequence with better efficacy in correcting the SCD mutation. For the effective delivery of the Cas9 system, anelectroporation method has been used with an Adeno-associated virus (AAV)-6 viral vectors (Cao and Vickers, 2021). Recently various clinical trials have been investigating the future scope for applying the technology by avoiding its negative consequences which might cause a random off-target effect along with subtherapeutic efficiency (Kanter et al., 2021). Approaches have been made for manufacturing the clinical grade guides cost-effectively which include the Cas9 and RNA proteins.
Nanotechnology
Nanotechnology has the potential to plays a major role in the advancement of new treatments for Sickle Cell anaemia. Several approaches are being explored, including the use of nanoparticles for drug delivery, the development of nanosensors for disease monitoring, and the use of nanomaterials for tissue engineering (Jiaoet al., 2021). However, rapid progress and development have been observed in the area of biomedicine and blood substitute or artificial bloodin nanotechnology which shows promising application in the emergency treatment of sickle cell anaemia. Recent advances in artificial blood development have developed various components which include immune response components (white blood cell substitutes) gas carrier components (erythrocyte substitutes), and hemostasis-responsive components (platelet substitutes) (DeBaunet al., 2020). The RBC substitutes produced by using nanotechnology can be used as an emergency treatment for sickle cell anaemia.
The RBC substitutes can be used as an alternative approach for blood transfusion in case of sickle cell anaemia. The advantage of these substitutes lies in the context that it has no surface antigens and is more convenient for storage compared to natural blood. This functions very effectively as haemoglobin which can be effective in correcting the oxygen-carrying problem in sickle cell anaemia (Kassim and Leonard, 2022). These substitutes can be significantly used in the case of "Hemorrhagic shock" which prevalently occurs in anaemia. These substitutes are produced by modifying the haemoglobin by crosslinking the two alpha helix units during the maintenance oftetramer conformation. These techniques produce the high oxygen-carrying capacity of the substitutes (Henryet al., 2021).
One potential use of nanoparticles is in the targeted delivery of drugs to specific cells or tissues in the body. Currently, researchers are exploring the use of nanoparticles coated with antibodies that target specific molecules on the surface of sickle cells, allowing drugs to be delivered directly to these cells (Brandow et al., 2020).This approach may increase the efficacy of drugs and reduce side effects. Nanosensors are also being developed for the real-time monitoring of disease progression and treatment response. These sensors can detect specific biomolecules or cellular changes associated with Sickle Cellanaemia and transmit this information to a monitoring device or healthcare provider (Wonkam, 2023). This can help to improve disease management and prevent complications.Another potential use of nanotechnology is in tissue engineering. Researchers are exploring the use of nanomaterials to create scaffolds for the growth of new tissues, such as bone marrow or blood vessels. This approach may provide a new option for the treatment of Sickle Cell anaemia, as it could allow for the creation of healthy bone marrow or blood vessels to replace damaged tissue (Magnaniet al., 2020).
Others
Various advances have been made in the early detection of sickle cell anaemia that used smartphone technology for the early identification of the disease. The conventional diagnostic methods which are based on laboratory technologies are extremely complex and time-consuming, thus advances have been made to differentiate between normal and sickle red blood cells using Optical microscope-based counting and classification (Weiss et al., 2021). The technology can be used to provide a home-based diagnosis and monitoring in the areas where resources are limited. Thus a smartphone-based image acquisition method has been developed forcapturing "RBC images" in patients suffering from sickle cell anaemia and showinghypoxia conditions. An advancedcomputer algorithm has been utilised to distinguish between the blood samples of the patient.
General Conclusion
In individuals with sickle cell anaemia, a single amino acid substitution occurs in one of the protein subunits of haemoglobin. Instead of a normal glutamic acid, the valine is present within its place. This results in a change in the structure of haemoglobin and causes the protein to aggregate and form long, rigid, sickle-shaped structures in the red blood cells. These sickle-shaped red blood cells are less flexible and have a shorter lifespan, leading to a reduced oxygen-carrying capacity of the blood, and an increased risk of blood vessel blockages, which can cause severe pain and other complications.Gene therapies involve modifying the genetic code to correct the mutation that causes Sickle Cell anaemia. The CRISPR approach is to use a viral vector to deliver a functional copy of the beta-globin gene to the bone marrow stem cells, which can then produce healthy red blood cells. Another approach is to use gene editing technologies such as CRISPR to correct the mutation directly. While these approaches are still in the early stages of development, they show promising results in preclinical studies.
References
- Abdulwahab, H., Aljishi, M., Sultan, A., Al-Kafaji, G., Sridharan, K., Bakhiet, M. and Taha, S., 2021. Whole blood transcriptomic analysis reveals PLSCR4 as a potential marker for vaso-occlusive crises in sickle cell disease.Scientific Reports,11(1), pp.1-9.
- Adio, W.S., Christopher, F.C., Adesola, R.O. and Mary, F.I., 2022. Current trends in the treatment of sickle cellanaemia.World News of Natural Sciences,43, pp.60-75.
- Al-Janabi, G.G., Al-Fahham, A.A. and kareem Mohammed, R., 2021. Pathophysiology of Sickle Cellanaemia.Indian Journal of Forensic Medicine & Toxicology,15(2), pp.2037-2043.
- Bhaskar, L.V., 2019. Factor V Leiden and prothrombin G20210A mutations and risk of vaso-occlusive complications in sickle cell disease: A meta-analysis through the lens of nephrology.Journal of Nephropharmacology,8(2), pp.e16-e16.
- Brandow, A.M., Carroll, C.P., Creary, S., Edwards-Elliott, R., Glassberg, J., Hurley, R.W., Kutlar, A., Seisa, M., Stinson, J., Strouse, J.J. and Yusuf, F., 2020. American Society of Hematology 2020 guidelines for sickle cell disease: management of acute and chronic pain.Blood advances,4(12), pp.2656-2701.
- Cao, H. and Vickers, M.A., 2021. Oxidative stress, malaria, sickle cell disease, and innate immunity.Trends in Immunology,42(10), pp.849-851.
- Cao, H. and Vickers, M.A., 2021. Oxidative stress, malaria, sickle cell disease, and innate immunity.Trends in Immunology,42(10), pp.849-851.
- Carden, M.A., Fasano, R.M. and Meier, E.R., 2020. Not all red cells sickle the same: contributions of the reticulocyte to disease pathology in sickle cellanaemia.Blood reviews,40, p.100637.
- Chu, S.H., Packer, M.S., Olins, J.R., Lung, G., Cheng, L.I., Liquori, A., Lee, C., Marshall, J., Yan, B., Decker, J. and Gantzer, B., 2020. A novel base editing approach to directly edit the causative mutation in sickle cell disease.Mol. Ther,28, p.808.
- DeBaun, M.R., Jordan, L.C., King, A.A., Schatz, J., Vichinsky, E., Fox, C.K., McKinstry, R.C., Telfer, P., Kraut, M.A., Daraz, L. and Kirkham, F.J., 2020. American Society of Hematology 2020 guidelines for sickle cell disease: prevention, diagnosis, and treatment of cerebrovascular disease in children and adults.Blood advances,4(8), pp.1554-1588.
- Demirci, S., Leonard, A., Haro-Mora, J.J., Uchida, N. and Tisdale, J.F., 2019. CRISPR/Cas9 for sickle cell disease: applications, future possibilities, and challenges.Cell Biology and Translational Medicine, Volume 5: Stem Cells: Translational Science to Therapy, pp.37-52.
- Ekambaram, R. and Rajasekaran, I., 2019. Who power sickle cell disease: Carbon domain analysis tells all because of design in protein 3D arbitrary internal carbon domain (COD) arrangement.Int J Mol Biol Open Access,4(3), pp.85-88.
- El Hoss, S., Cochet, S., Godard, A., Yan, H., Dussiot, M., Frati, G., Boutonnat-Faucher, B., Laurance, S., Renaud, O., Joseph, L. and Miccio, A., 2020. Fetalhaemoglobin rescues ineffective erythropoiesis in sickle cell disease.Blood,136, pp.14-15.
- Germino-Watnick, P., Hinds, M., Le, A., Chu, R., Liu, X. and Uchida, N., 2022. Hematopoietic stem cell gene-addition/editing therapy in sickle cell disease.Cells,11(11), p.1843.
- Haro-Mora, J.J., Uchida, N., Demirci, S., Wang, Q., Zou, J. and Tisdale, J.F., 2020. Biallelic correction of sickle cell disease-derived induced pluripotent stem cells (iPSCs) confirmed at the protein level through serum-free iPS-sac/erythroid differentiation.Stem Cells Translational Medicine,9(5), pp.590-602.
- Henry, E.R., Metaferia, B., Li, Q., Harper, J., Best, R.B., Glass, K.E., Cellmer, T., Dunkelberger, E.B., Conrey, A., Thein, S.L. and Bunn, H.F., 2021. Treatment of sickle cell disease by increasing oxygen affinity of haemoglobin.Blood,138(13), pp.1172-1181.
- Ji, K., 2020. Correction of the Sickle Cell Mutation Through Base and Prime Editing in Hematopoietic Stem Cells.
- Jiao, B., Basu, A., Roth, J., Bender, M., Rovira, I., Clemons, T., Quach, D., Ramsey, S. and Devine, B., 2021. The use of cost-effectiveness analysis in sickle cell disease: a critical review of the literature.Pharmacoeconomics,39(11), pp.1225-1241.
- Kanter, J., Liem, R.I., Bernaudin, F., Bolaños-Meade, J., Fitzhugh, C.D., Hankins, J.S., Murad, M.H., Panepinto, J.A., Rondelli, D., Shenoy, S. and Wagner, J., 2021. American Society of Hematology 2021 guidelines for sickle cell disease: stem cell transplantation.Blood Advances,5(18), pp.3668-3689.
- Kassim, A.A. and Leonard, A., 2022. Debating the Future of Sickle Cell Disease Curative Therapy: Haploidentical Hematopoietic Stem Cell Transplantation vs. Gene Therapy.Journal of Clinical Medicine,11(16), p.4775.
- Magnani, A., Pondarré, C., Bouazza, N., Magalon, J., Miccio, A., Six, E., Roudaut, C., Arnaud, C., Kamdem, A., Touzot, F. and Gabrion, A., 2020. Extensive multilineage analysis in patients with mixed chimerism after allogeneic transplantation for sickle cell disease: insight into hematopoiesis and engraftment thresholds for gene therapy.Haematologica,105(5), p.1240.
- Martins, G. and Galamba, N., 2023. Protein Aggregation-Inhibition: A Therapeutic Route from Parkinson's Disease to Sickle Cellanaemia.
- Menzel, S. and Thein, S.L., 2019. Genetic modifiers of fetal haemoglobin in sickle cell disease.Molecular Diagnosis & Therapy,23(2), pp.235-244.
- Neal, T., 2021. Smoothie Recipes To Improve Hydration And Reduce The Risk Of Pain Crisis In Children With Sickle Cellanaemia.
- Nkya, S., Mwita, L., Mgaya, J., Kumburu, H., Van Zwetselaar, M., Menzel, S., Mazandu, G.K., Sangeda, R., Chimusa, E. and Makani, J., 2020. Identifying genetic variants and pathways associated with extreme levels of fetal haemoglobin in sickle cell disease in Tanzania.BMC Medical Genetics,21, pp.1-12.
- Obeagu, E.I., Muhimbura, E., Kagenderezo, B.P., Uwakwe, O.S. and Nakyeyune, S., 2022. An Update on Interferon Gamma and C Reactive Proteins in Sickle Cell Anaemia Crisis.J Biomed Sci,11(10), p.84.
- Penkert, R.R., Chandramouli, S., Dormitzer, P.R., Settembre, E.C., Sealy, R.E., Wong, S., Young, N.S., Sun, Y., Tang, L., Cotton, A. and Dowdy, J., 2021. Novel Surrogate Neutralizing Assay Supports Parvovirus B19 Vaccine Development for Children with Sickle Cell Disease.Vaccines,9(8), p.860.
- Quagliano, A., Acevedo, D., Hardigan, P. and Prasad, S., 2022. Using Clustered Regularly Interspaced Short Palindromic Repeats gene editing to induce permanent expression of fetalhaemoglobin in β-thalassemia and sickle cell disease: A comparative meta-analysis.Frontiers in Medicine,9.
- Sundd, P., Gladwin, M.T. and Novelli, E.M., 2019. Pathophysiology of sickle cell disease.Annual review of pathology: mechanisms of disease,14, pp.263-292.
- Tisdale, J.F., Thein, S.L. and Eaton, W.A., 2020. Treating sickle cellanaemia.Science,367(6483), pp.1198-1199.
- Uchida, N., Drysdale, C.M., Nassehi, T., Gamer, J., Yapundich, M., DiNicola, J., Shibata, Y., Hinds, M., Gudmundsdottir, B., Haro-Mora, J.J. and Demirci, S., 2021. Cas9 protein delivery non-integrating lentiviral vectors for gene correction in sickle cell disease.Molecular Therapy-Methods & Clinical Development,21, pp.121-132.
- Vendrame, F., Olops, L., Saad, S.T.O., Costa, F.F. and Fertrin, K.Y., 2019. Hypocholesterolemia and dysregulated production of angiopoietin-like proteins in sickle cellanaemia patients.Cytokine,120, pp.88-91.
- Ware, R.E. and Dertinger, S.D., 2021. Absence of hydroxyurea?induced mutational effects supports higher utilisation for the treatment of sickle cell anaemia.British Journal of Haematology,194(2), pp.252-266.
- Weiss, S., Yang, S., Zhang, S., David, M., Lanzkron, S.M. and Eakin, M., 2021. The telemedicine experience for individuals with sickle cell disease.Blood,138, p.1893.
- Wonkam, A., 2023. The future of sickle cell disease therapeutics rests in genomics.Disease Models & Mechanisms,16(2), p.dmm049765.
- Yousif, T.Y.E., 2022. Impact of Abnormal Leukocyte Count in the Pathophysiology of Sickle Cellanaemia.Journal of Blood Medicine, pp.673-679.